Lattice Field Theory

Lattice QCD (QCD is a field theory) is a non-perturbative approach to compute physical observables from first principles. It can be viewed as way of computing path integral correlation functions numerically and is based on Monte Carlo simulations.
The appeal is not only that it is a method from first principals, but also that it is possible to access information that cannot be obtained by experiments. Simple results like particle spectra are always a starting point before tackling more complicated observables like distribution amplitudes or form factors that may provide insights into fundamental parameters like CKM matrix elements.
Wikipedia claims: "Lattice QCD has also been proposed as a possible benchmark for high-performance computing."
For queries about this topic, contact Dirk Broemmel.
View the calendar of events relating to this topic.
Projects
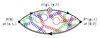
B-meson coupling with relativistic heavy quarks
Jonathan Flynn (Investigator), Ben Samways, Dirk Broemmel, Patrick Fritzsch
We non-perturbatively compute the coupling between B* and B pi meson states relying on relativistic heavy quarks and domain wall light fermions. The coupling is of importance for an effective description of hadronic heavy meson decays.

Hadronic structure on the computer
Jonathan Flynn (Investigator), Dirk Broemmel, Thomas Rae, Ben Samways
In experiments at the Large Hadron Collider (LHC) at CERN, Geneva, the interactions that occur between the colliding particles (protons in this case) can be factorised into a simple scattering between two constituent particles, called quarks, followed by a hadronisation process, which describes the dynamics of forming the bound proton states. Quarks are particles within the proton that bind to form composite particles (hadrons) such as a proton. The scattering process can be computed relatively easily, but hadronisation is intrinsically non-perturbative and hard to calculate. Lattice QCD (computer simulation of QCD on a discrete space-time lattice) provides our only known first-principles and systematically-improvable method to address problems like hadronisation. This project uses Iridis to extract parton distribution amplitudes which are experimentally inaccessible, but needed to describe the quark structure of hadrons.
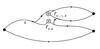
Kaon to two pion decays in lattice QCD
Jonathan Flynn (Investigator), Elaine Goode, Dirk Broemmel
We calculate kaon decay amplitudes on the lattice so we may compare the Standard Model to experiment.
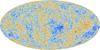
Lattice Holographic Cosmology
Andreas Juttner (Investigator), Matthew Mostert
This project will aim to develop new theoretical field methods and massively parallel computational algorithms to be utilised on both new computational architectures (e.g. Intel Xeon Phi) and existing high performance computers (HPCs).
The ultimate goal is to make predictions for the power spectrum and non-gaussianties of the CMB which would then be falsifiable by comparison to the Planck and WMAP data.
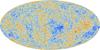
Lattice Holographic Cosmology
This project will aim to develop new theoretical field methods and massively parallel computational algorithms to be utilised on both new computational architectures (e.g. Intel Xeon Phi) and existing high performance computers (HPCs).
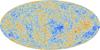
Lattice Holographic Cosmology
This project will aim to develop new theoretical field methods and massively parallel computational algorithms to be utilised on both new computational architectures (e.g. Intel Xeon Phi) and existing high performance computers (HPCs).
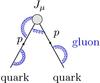
Non-Perturbative Renormalisation on the Lattice
Jonathan Flynn (Investigator), Dirk Broemmel, Thomas Rae
In this project we compute renormalisation factors for various physical observables in a non-perturbative lattice framework. Renormalisation hereby arises due to a fundamental scale dependence of the physical processes.
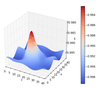
Precision study of critical slowing down in lattice simulations of the CP^{N-1} model
Jonathan Flynn, Andreas Juttner (Investigators), Andrew Lawson
This project involves the study of critical slowing down (CSD): a property that may arise when taking measurements in Monte Carlo simulations. In order to study and quantify this phenomenon we have performed extensive simulations of the CP^{N-1} model. By studying the properties of the Monte Carlo algorithms in this model, we hope to make algorithmic improvements that can then be employed in simulations of physical quantum field theories, such as in lattice quantum chromodynamics (lattice QCD).
pyQCD
Matthew Spraggs
A basic Python package to perform coarse lattice QCD simulations on desktop and workstation computers.

Towards Exascale computing in particle physics
Andreas Juttner, Jonathan Flynn (Investigators), James Harrison
Lattice QCD
People

Professor, Physics & Astronomy (FPAS)

Reader, Physics & Astronomy (FPAS)

Research Fellow, Physics & Astronomy (FPAS)

Postgraduate Research Student, Physics & Astronomy (FPAS)

Postgraduate Research Student, Engineering Sciences (FEE)

Postgraduate Research Student, Physics & Astronomy (FPAS)

Postgraduate Research Student, Physics & Astronomy (FPAS)

Postgraduate Research Student, Engineering Sciences (FEE)

Postgraduate Research Student, Electronics and Computer Science (FPAS)

Postgraduate Research Student, Physics & Astronomy (FPAS)

Postgraduate Research Student, Physics & Astronomy (FPAS)

Postgraduate Research Student, Electronics and Computer Science (FPAS)

Administrative Staff, Research and Innovation Services